Quantum communication advances on fiber networks
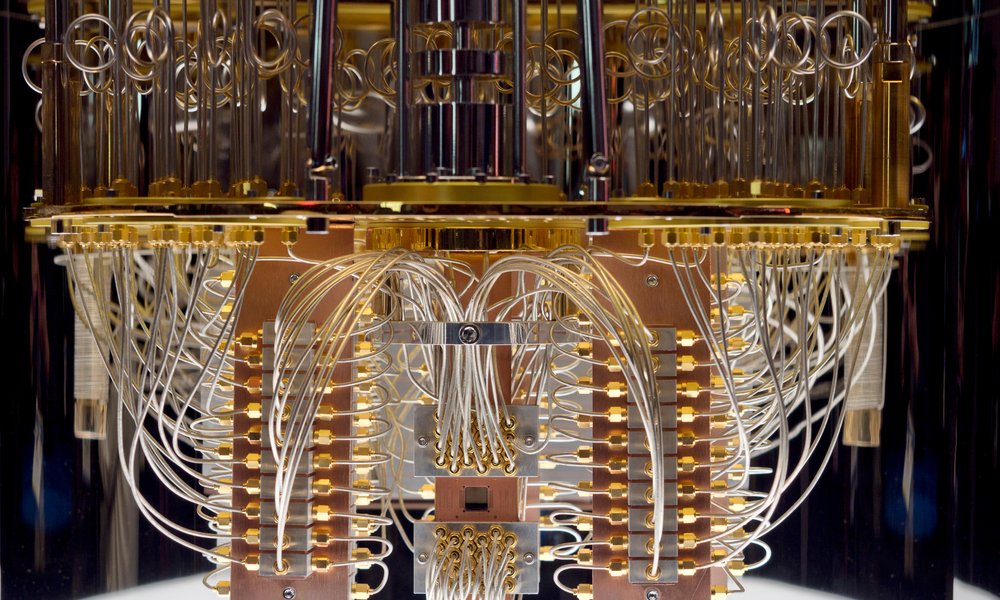
Quantum communication advances on fiber networks
The universities of Bristol and Cambridge in the UK and Deutsche Telekom in Germany have announced separate advances in quantum communications over classical fiber networks.
In the case of the UK, researchers demonstrated a videoconference, the transfer of encrypted medical data and secure remote access to a distributed data center between the two universities, which represents a distance of 410 kilometers.
They claimed it was the first time that “a long-distance network, encompassing different quantum-secure technologies such as entanglement distribution, has been successfully demonstrated.” (Quantum entanglement involves coupling qubits, which are the basic unit of quantum information, so that measuring one instantly influences the other or others it is entangled with.)
For Deutsche Telekom, progress came in the form of sustaining the high-fidelity (99%) transmission of entangled protons over a period of 17 days.
It is the latest progress by researchers and telecom operators, including not only Deutsche Telekom, but also BT, China Telecom, NTT, SK Telecom and Vodafone, to secure communications and protect data from future quantum computers that could break the public-key cryptography that protects today’s IT systems from hackers.
There is debate about when a so-called cryptographically-relevant quantum computer (CRQC) will be able to break today’s cryptography systems. And although there is agreement that it is still several years away, it is getting closer, according to the Global Risk Institute’s (GRI) latest Quantum Threat Timeline report.
“Today’s quantum processors are still far from being CRQCs, but the technology is advancing, with no known fundamental barrier, thus making a real CRQC a matter of 'when' rather than 'if.'” The GRI bases its reports on the insights of 32 global experts.
The most 'optimistic' interpretation of the responses (i.e. those who think it likely) resulted in a 34% estimate of a CRQC being developed within a decade and around 14% within 5 years. In 2023, the figures were 31% and 11% respectively. Even the most “pessimistic” interpretation gives a 19% average likelihood estimate of a disruptive quantum threat in the next 10 years, according to the GRI.
Deutsche Telekom makes headway on quantum
Matheus Sena, researcher at Deutsche Telekom's T-Labs, explained in emailed responses to questions from Inform, the operator's work on quantum networks and why there will be need for standardization.
- What do you see as being the main uses of entanglement-based networks? And how widespread do you expect them to come?
Entanglement is considered a fundamental resource for quantum networks, much like bandwidth is for classical networks. Its applications go far beyond the commonly cited use case of secure communication, making it central to the development of the quantum internet.
Some key use cases include:
- Security: Entanglement enables quantum key distribution (QKD), allowing the exchange of cryptographic keys that are secure against any attack, including those by quantum computers.
- Distributed Quantum Computing: In the future, entanglement will be used to interconnect quantum computers, allowing them to work together and share quantum information across long distances.
- Sensing: Quantum entanglement can boost the sensitivity and precision of sensors, improving measurements of physical quantities like magnetic fields —particularly relevant in industrial IoT environments.
- Time Synchronization: Entangled photons can help synchronize clocks between distant network nodes with extremely high precision, which could be especially valuable in satellite networks or timing infrastructures.
- Random Number Generation: Quantum processes based on entanglement provide true randomness, which is essential for secure cryptography and simulations.
These applications demonstrate the broad potential of entanglement as a technology enabler. That’s why we view it as a strategic resource for the future of communication networks, and one that will likely become increasingly important as quantum technologies mature and scale.
- What are the challenges of integrating an entanglement-based network with an existing commercial fiber network? How did Deutsche Telekom overcome the difficulties?
One of the main challenges is that field-deployed optical fibers are subject to a variety of external influences. Even slight mechanical disturbances - such as someone touching the fiber, vibrations from cooling fans in data centers, or routine maintenance by technicians - can disrupt the delicate quantum state of entangled photons. These disturbances can affect polarization, which is critical for preserving entanglement.
To overcome this, we employed an automatic polarization control system (APC) which was developed by Qunnect for this purpose. This system continuously monitors changes in polarization within the fiber and applies active corrections in real time. It’s a key technology that allows quantum systems to operate reliably over existing fiber infrastructure.
Another challenge is that entangled photons are extremely sensitive to noise—particularly from classical data signals transmitted in the same fiber. However, if we want quantum systems to coexist with classical networks, we need efficient multiplexing strategies. To address this, we used entangled photons at 1324 nm, which is spectrally well-separated from the classical transmission in the C-band (around 1550 nm). This spectral separation minimizes noise interference and also reduces dispersion effects, making the transmission of entangled photons more stable over longer distances.
- Are there constraints on the sort of fiber network that can be used for a ‘quantum internet’? If so, what are they?
The fiber itself isn’t fundamentally different - it’s typically the same single-mode fiber that’s already deployed worldwide. However, there are specific constraints and requirements when using this infrastructure for quantum communication. One key limitation is loss. In classical networks, signal loss can be compensated with amplifiers, but in quantum networks, amplifying a qubit would destroy its quantum state. This means the fiber used for entanglement distribution should have as low attenuation as possible. In practice, however, real-world fiber networks are not perfect. They’re more like a mosaic - composed of fiber segments, splices, connectors, and various passive components like filters used for wavelength multiplexing. Each of these introduces a bit of loss, and over long distances, these losses add up significantly. As a result, the longer the link, the more difficult it becomes to successfully transmit and detect entangled photons on the other end. That’s why optimizing the physical path and minimizing insertion losses is critical when adapting existing fiber networks for quantum communication.
- Is there a need for the telecoms industry to work on hardware / software compliance between future quantum networks?
Yes, definitely. While we may continue to use a layered model like the OSI framework to describe quantum networks, the functionalities within each layer will be fundamentally different. For example, in the physical layer, we’ll see components like entangled photon sources and quantum memories working to establish entanglement between adjacent nodes. The network layer will be responsible for managing and maintaining end-to-end entanglement, and the transport layer will handle the reliable transmission of quantum states - or qubits - across the network.
To make this architecture interoperable and scalable, we need standardized interfaces between software and hardware. This includes synchronization protocols, memory access mechanisms, and entanglement management across diverse platforms and vendors. A key challenge remains the maturity of quantum hardware. Many essential components - like quantum memories - are still in development and often limited to lab settings. To build viable quantum networks, we need robust, telecom-compatible hardware that operates under realistic conditions, ideally at room temperature. In our lab, for example, we’re already moving towards testing rack-mounted, field-deployable systems, which represent an important step toward bridging experimental setups and real-world telecom environments. Collaboration across the telecom and quantum industries will be essential to ensure compatibility, compliance, and interoperability as quantum networks begin to take shape.
- Why did you test polarization entanglement? And are there are choices available to the telecoms industry)
Yes, there are multiple ways to encode entanglement—such as frequency-bin and time-bin entanglement—each with its own characteristics. We chose polarization entanglement for several reasons. First, it is highly compatible with established quantum technologies, particularly those based on atoms and ions, which often interact with photons via their polarization. Second, polarization is easier to manipulate and analyze, requiring only relatively simple optical components like waveplates and polarizers. In contrast, time-bin entanglement typically relies on interferometric setups, which are more complex and can become significantly more expensive. This relative simplicity makes hardware development more practical and accessible, helping to bring polarization-based quantum communication one step closer to real-world deployment.
- How does your work fit with the work on QKD that Deutsche Telekom is undertaking as part of Nostradamus?
The recent demonstration of quantum entanglement represents a key milestone on the path toward a scalable quantum internet – and complements our work within the NOSTRADAMUS project perfectly. While NOSTRADAMUS focuses on quantum key distribution (QKD) using today’s available technology, typically involving trusted nodes, our entanglement-based research lays the foundation for the next generation: quantum repeaters that will enable long-distance quantum-secure communication without the need for trusted nodes. This paves the way for true end-to-end encryption based on quantum mechanical principles. Both efforts contribute to a shared objective – building a secure and high-performance quantum communication infrastructure in Europe. We see not only strong technological synergies, but also the opportunity to help shape international standards and reinforce Europe’s digital sovereignty.